November 2024, Vol. 251, No. 11
Features
Optimizing Gas Analysis in Carbon Capture, Utilization and Storage
Ruth Lindley, Emerson, Falkirk, Scotland, U.K.
As the global demand for energy grows, so too does the need to transition toward sustainable energy sources. For many process manufacturers, carbon capture, utilization and storage (CCUS) is a central part of their efforts to reduce emissions of one of the most prevalent greenhouse gases (GHGs), carbon dioxide (CO2).
With CCUS, CO2 emissions are captured at the source, and then they are either stored or used in other industrial processes — both cases often require transportation from the production site.
Carbon capture has been around for many years, but it gained traction in recent years as technologies evolved to allow CO2 to be removed and sequestered indefinitely. Today, CCUS has the potential to remove up to 99% of an industrial plant’s carbon emissions, making a significant and positive impact to GHG emissions and climate change.
Although the ability to capture and store CO2 emissions underground has significant benefits, it is not without its challenges. The financial viability of a CCUS operation is a particular concern because capturing CO2 can decrease a plant’s efficiency and increase its energy use, while adding expense. These additional costs can ultimately render a CCUS project uneconomical.
Transporting CO2 is also expensive, because the CO2 must be compressed, requiring significant energy input, and pipelines must be able to withstand the high pressures required for transport. Any impurities in the CO2 stream, such as moisture and acidic gases, can lead to accelerated pipeline corrosion.
As with many industrial processes, the ability to accurately measure the concentration of bulk gas — in this case, CO2 — along with any impurities within it, provides significant benefits. These types of measurements can be used to optimize the processes producing CO2, minimize the risk of corrosion in pipelines and ensure product quality at custody transfer points.
Gas Analysis
The CCUS market encompasses wide-ranging applications, with different challenges when it comes to gas analysis. No two CCUS applications are the same, and therefore, each requires a tailored approach to best meet gas analysis requirements.
Typical instruments used in these applications include gas chromatographs (GCs), novel laser-based analyzers incorporating both quantum cascade lasers (QCL) and tunable diode lasers (TDL), and continuous process gas analyzers (PGAs) incorporating traditional technologies, such as non-dispersive infrared (NDIR) and thermal conductivity detectors (TCD).
Each type of instrument provides different advantages and drawbacks in CCUS applications, and they are often complementary, with an installation requiring multiple product types to cover the range of required measurements. Gas components present in CCUS applications and the analysis technologies available to detect them (Table 1).
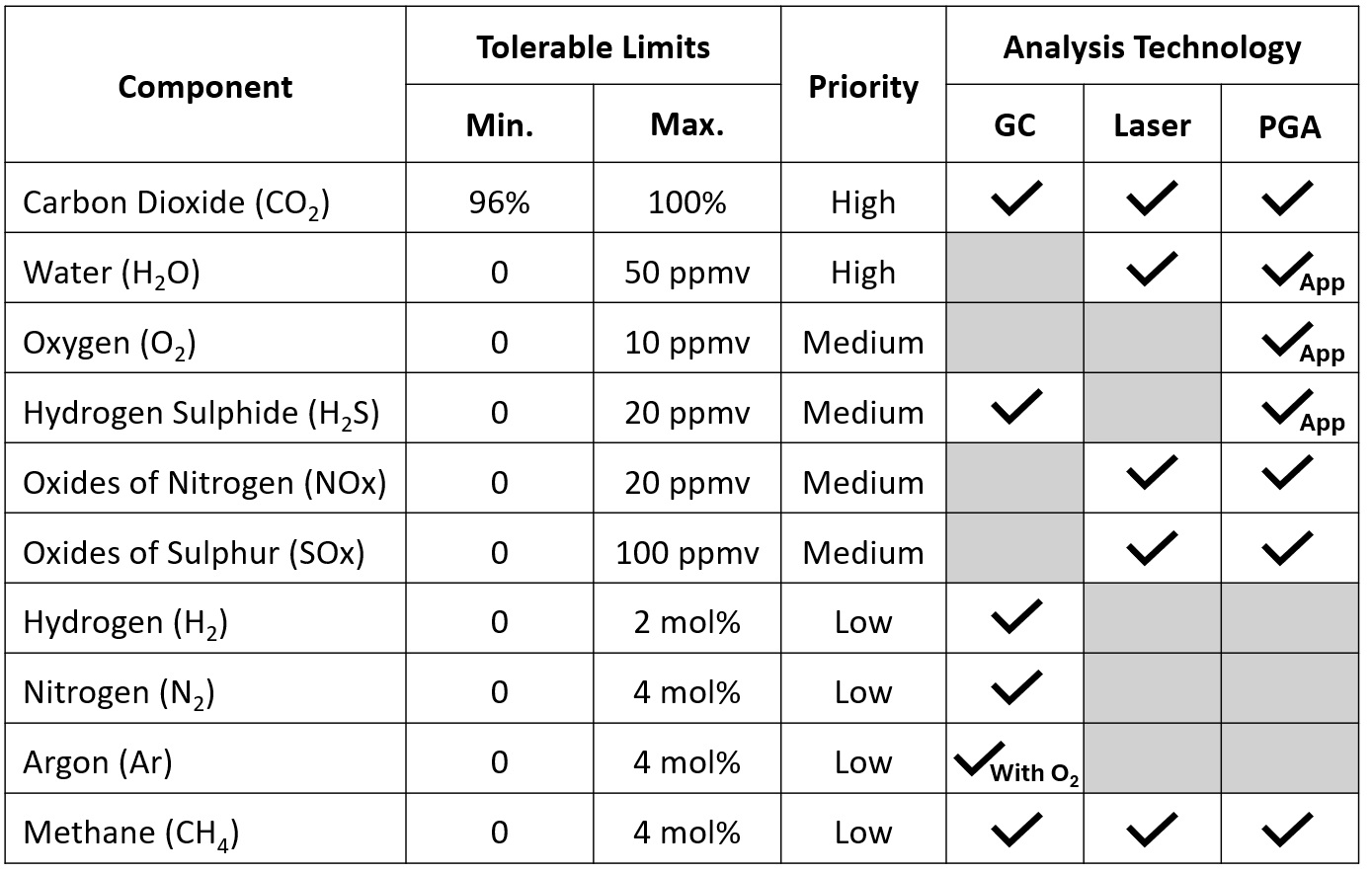
The selection of a gas analyzer depends on more than the gases that it can detect, as it must also consider the number of gases that can be measured by a single system, precision and accuracy of the measurement, complexity of the installation, ongoing maintenance requirements, lifecycle costs and other factors.
GCs operate based on the chromatography principle of separation and detection. Analytical valves inject a sample into different flow paths, which consist of columns, valves and detectors. Columns separate the gas components from a homogenous sample, which are detected as they exit the column using either a thermal conductivity, flame ionization or flame photometric detector.
GCs can be configured to offer a wide range of measurements by optimizing the design of the flow path, and they have a broad measurement range, from parts per billion to percentage levels.
In CCUS applications, GCs are recommended for direct measurements of CO2, plus a range of impurities, including inert components such as hydrogen (H2), nitrogen and argon, which are typically not measured by spectroscopic techniques due to their very weak spectra or lack thereof. Hydrocarbons, carbon monoxide (CO), sulfur species and low levels of formaldehyde or formic acid can also be monitored.
GCs provide measurements with cycle times that are typically in minutes and provide the comprehensive composition analysis often required for quality or process monitoring purposes. Periodic calibrations are needed to maintain measurement repeatability, but these can be configured to run automatically.
Laser-based analyzers offer highly sensitive and selective measurements of a range of small, light gases, including CO2. The lasers are operated in rapid-pulse mode, recording thousands of spectra each second, which can be averaged to provide an excellent signal-to-noise ratio, very low detection limits and fast and continuous measurements.
Up to six lasers can be installed in a single analyzer, providing the capability to measure up to 10 components simultaneously.
Laser-based analyzers offer long lifetimes, due to their use of a stable solid-state source and lack of moving parts. The inherent stability of these laser-based measurements means that calibration frequencies can be reduced compared to traditional technologies such as NDIR and NDUV, reducing lifecycle costs.
However, the price of a laser-based analyzer is typically higher than a PGA analyzer incorporating traditional continuous gas analysis techniques. In CCUS applications, laser technology provides measurement of bulk CO2, along with impurities such as water, methane (CH4), nitrogen oxides and sulfur dioxide.
PGA Operation
PGAs can incorporate NDIR and TCD technologies, alongside paramagnetic oxygen (O2) measurements and electrochemical sensors. Some PGAs can make up to five measurements, and they can incorporate a range of different technologies to offer an optimum suite of measurements for a particular application.
PGAs offer a cost-effective and flexible continuous gas analyzer solution, with the ability to detect a range of different gases with wide measurement ranges, along with excellent sensitivity and selectivity. These analyzers often include moving parts, such as chopper wheels, which require periodic replacement, and they require more frequent calibrations than laser-based analyzers. PGA typically provides the broadest measurement coverage in CCUS applications, including bulk CO2 concentration.
The following examples describe the analytical solutions deployed for different CCUS applications, using GC, PGA and QCL technologies:
Case Study 1:
Enhanced gas recovery – A U.S.-based company was using CO2 for enhanced gas recovery by injecting it into a depleted reservoir to increase the production of natural gas from the well.
The CO2 was a byproduct of another operation, and the enhanced gas recovery process reduced the site’s carbon footprint and maximized the efficiency of the well. In this installation, which is shown schematically in Figure 1, the operating company needed to monitor CO2 both at the injection site and the production well to determine when optimum injection and recovery rates had been achieved.
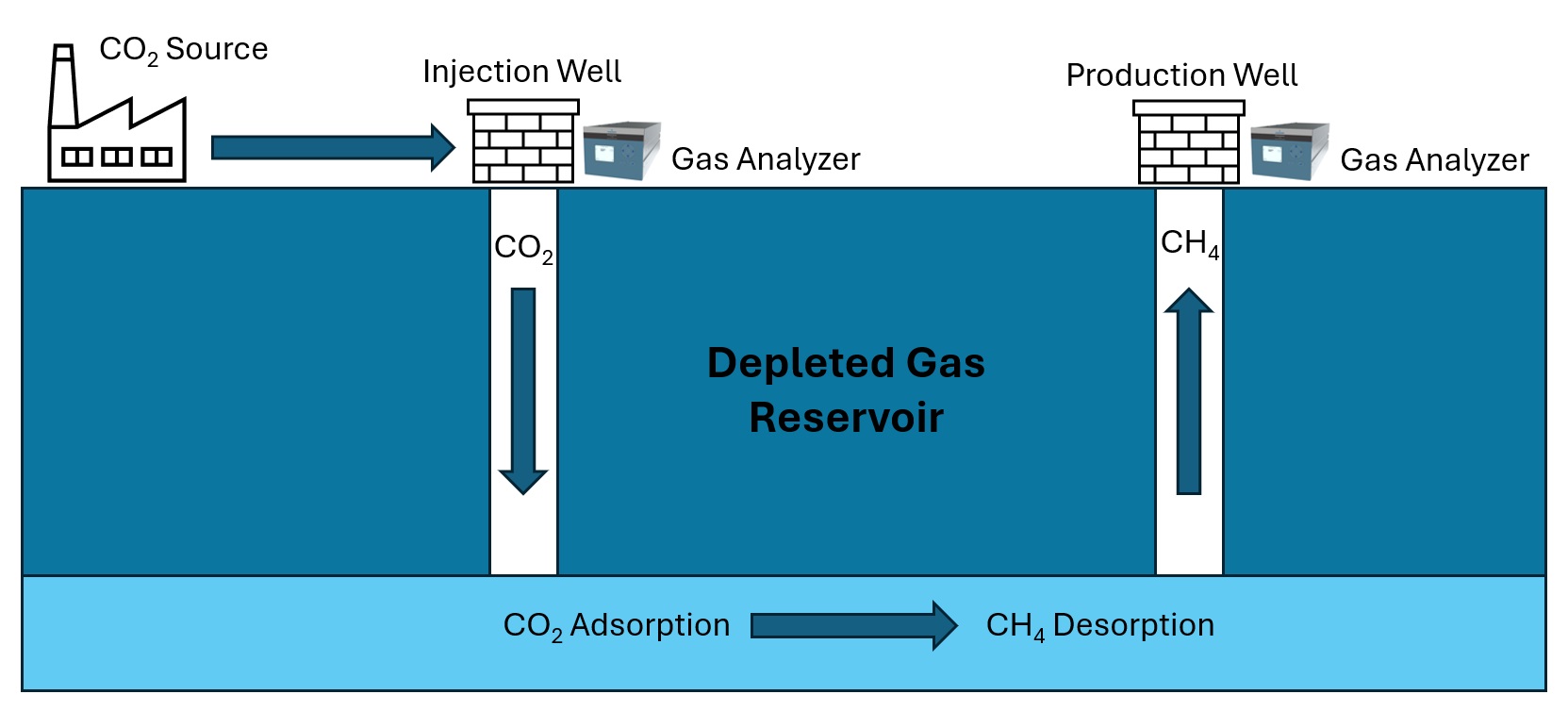
The company selected a QCL-based gas analyzer (Figure 2). The analyzer was configured to monitor CO2 over a range of 0%–100% with a detection limit of 0.25%, and it was selected based on speed-of-response, accuracy over a wide measurement range and quick delivery lead time.
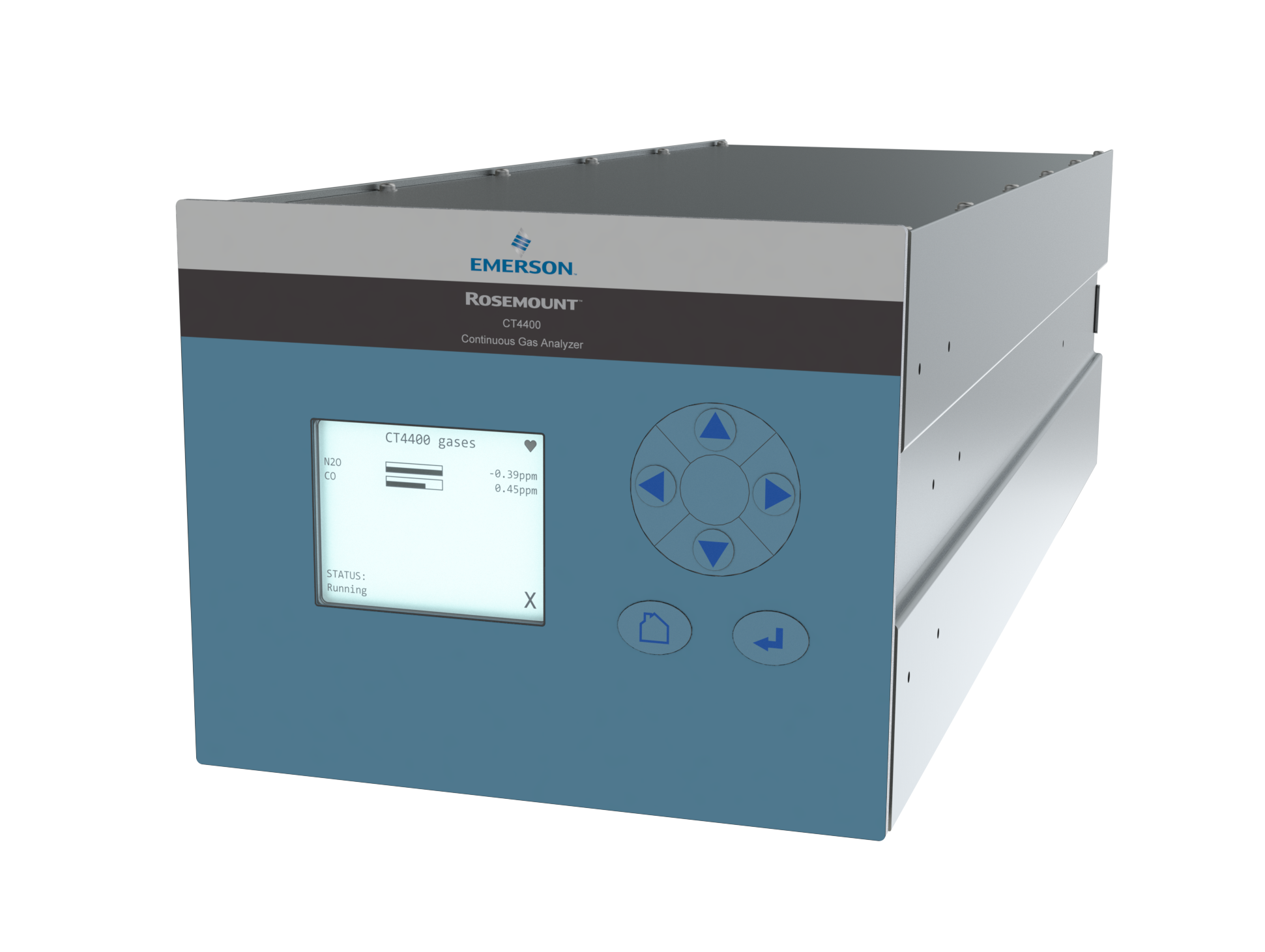
Case Study 2:
CO2 transportation – A U.S.-based company running a gas processing operation was producing CO2, which was subsequently transported from the site. Before transportation, impurities in the CO2 were measured to ensure quality standards were being met and to reduce the risk of damage to transportation equipment from the presence of contaminants.
The author’s company’s GC analyzerb (Figure 3) was selected for this application to monitor bulk CO2 concentration, along with inert components, including H2, nitrogen, O2 and helium.
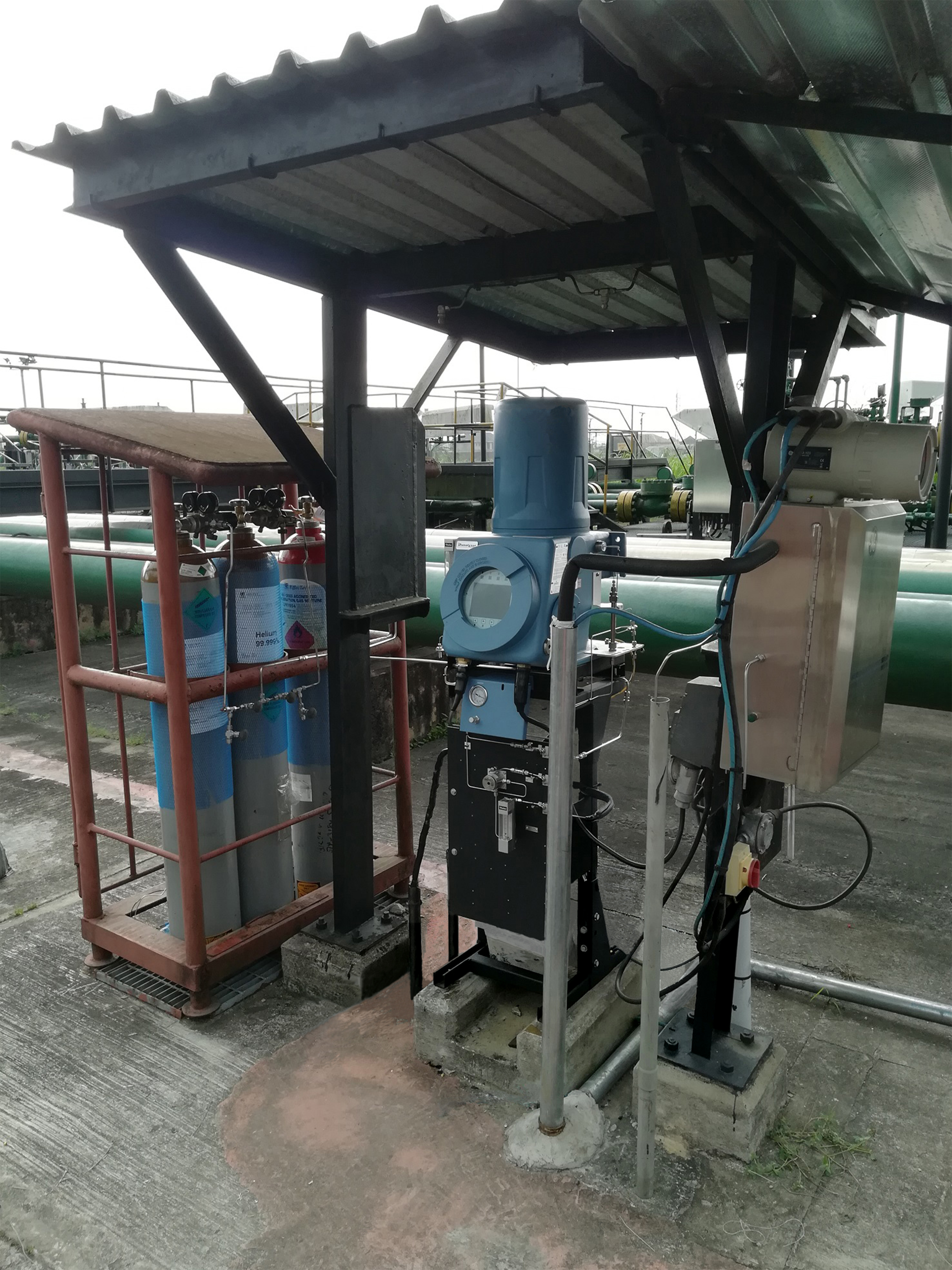
The GC has dual TCD detectors and provides measurements every three minutes. The measurement specification for the GC analyzerb (Table 2).
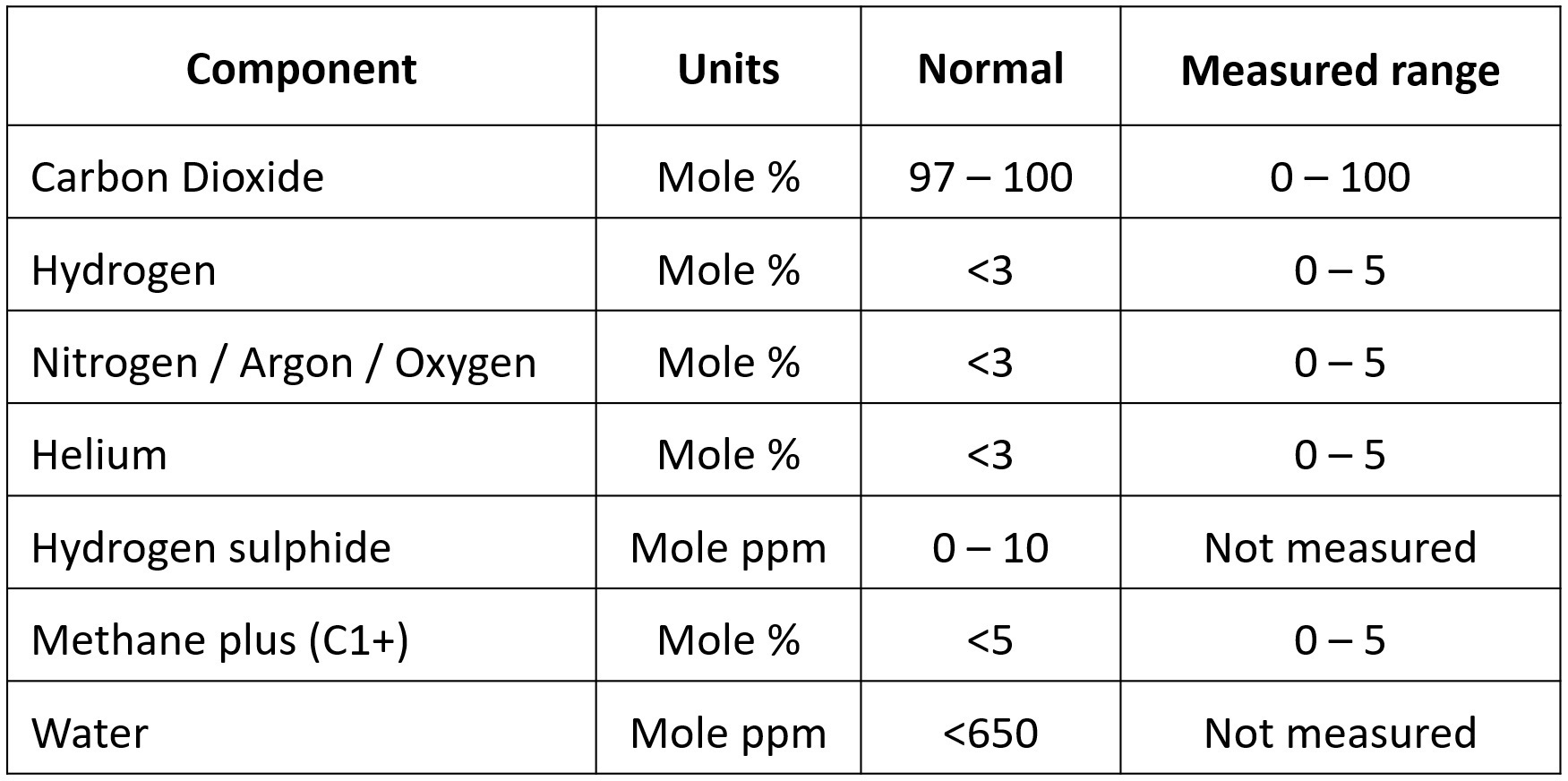
Case Study 3:
CO2 capture for blue H2 production – Blue H2 is created when CO2 emissions are captured and sequestered to make the H2 production process carbon neutral. A European company was using a cryogenic method to decarbonize their site by capturing more than 100,000 tpy of CO2, and it opted to use the author’s company’s PGA analyzerc to monitor the composition of flue gas from its steam methane reforming process to optimize efficiency.
Three analyzers (Figure 4) were configured to continuously monitor emissions of CO, CO2, CH4, H2 and O2 using a range of technologies, including NDIR, electrochemical and TCD.
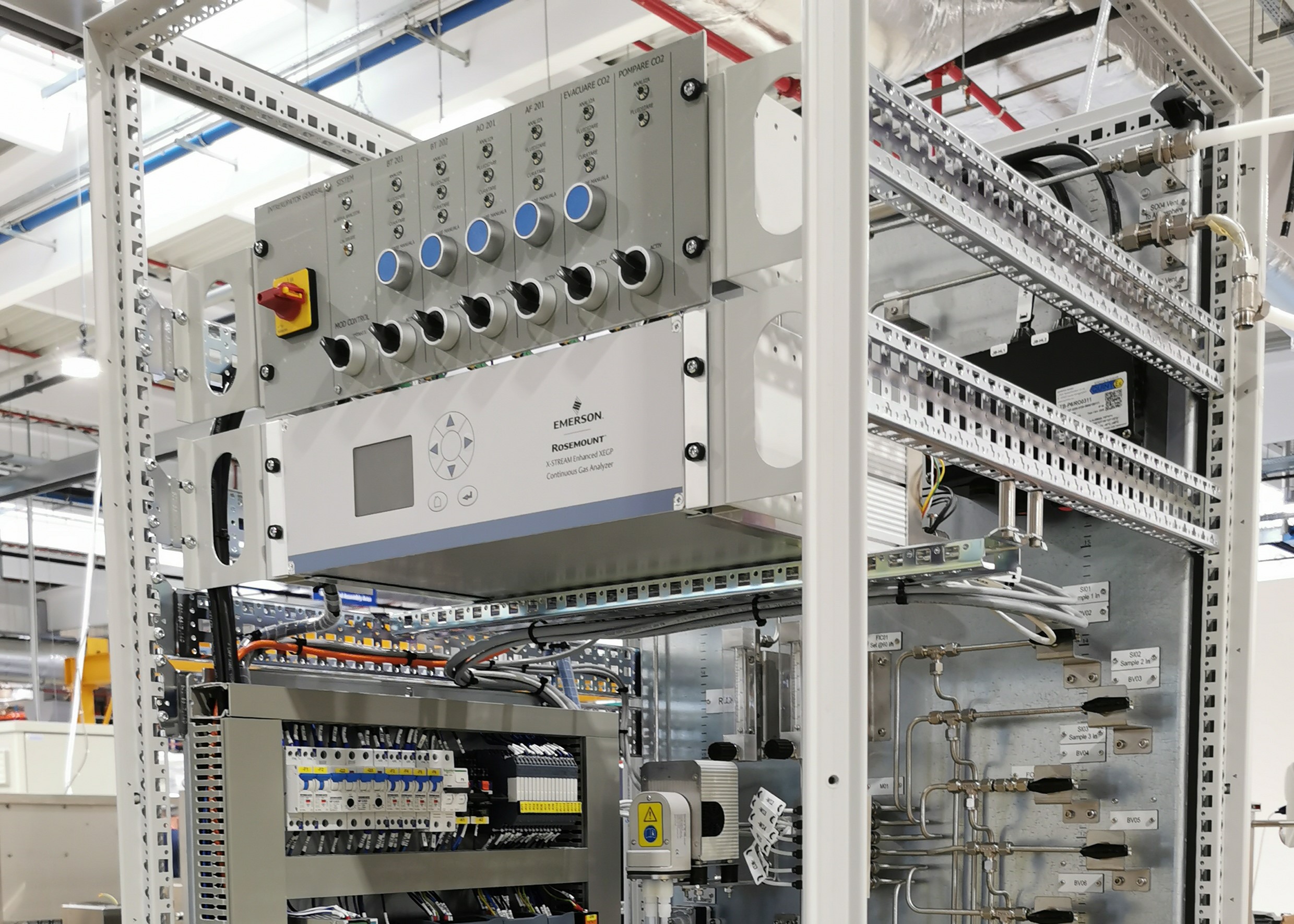
These analyzers were selected due to their versatile measurement capability, as well as offering a cost-effective, multiple gas analyzer solution. The specifications of the analyzers used in this installation can be seen in Table 3.
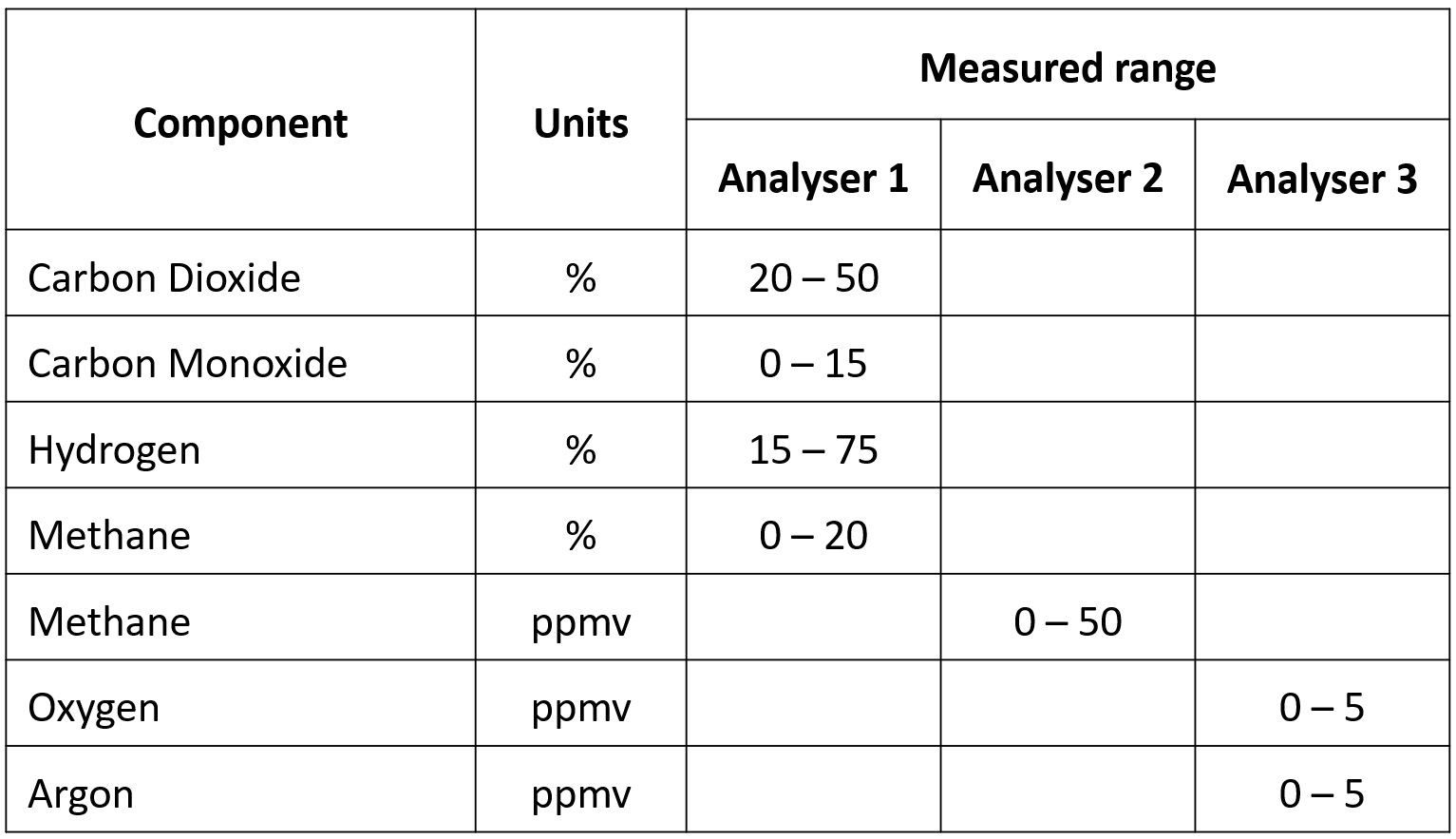
Takeaway
CCUS is a growing market as companies strive to reduce their carbon footprint, and it has a wide range of applications and measurement requirements. A flexible approach is required when specifying an analytical solution for a particular application, with different technologies offering differing strengths and measurement capabilities.
Certain suppliers, with a range of technologies and products on offer, can help an operating company develop an analytical package that is an ideal fit for their requirements.
Notes:
an Emerson Rosemount™ CT4400 QCL-based gas analyzer
b Emerson Rosemount™ 700XA GC analyzer
c Emerson Rosemount™ X-Stream XEGP analyzer
Author: Ruth Lindley is the director of Product Management for Rosemount Continuous Gas Analyzers at Emerson. She earned a PhD in experimental physical chemistry from the University of Bristol.
Comments