Capturing Waste Heat from Gas Compressor Stations

In view ensuring the efficient use of fossil fuel energy and to reduce overall carbon emissions, the possibilities of maximizing exhaust-heat recovery should be seriously considered. Gas turbines reject 50% or more of the energy in the fuel into the atmosphere through the exhaust systems.
Some of this energy can help make steam and provide heat to process plants or to generate electricity. Gas turbines utilized to drive gas compressors in pipeline service could benefit from the use of energy recovery systems to reach optimal emission and consumption figures.
The majority of compressor stations are in remote locations that lack adequate water supplies. For many jurisdictions, a steam system also requires the services of a certificated engineer 24 hours a day. Compressor stations operate remotely without a permanent staff on site, and the burden of this requirement adds substantially to the operating costs. A heat-recovery system for a compressor station, therefore, has to be based on a Rankine cycle using an organic fluid rather than steam.
The mechanical work output of the cycle can be used to generate electricity and increase the overall efficiency of the plant without adding to emissions. The electricity generated can provide revenue and, by displacing power generated from baseload power stations, contribute to an overall reduction in carbon emissions.
Organic Rankine Cycle
The elements of the organic Rankine cycle (ORC) consist of a heat exchanger in the exhaust system of the gas turbine, an expander turbine driving an electric generator, a water-cooled or air-cooled condenser, a working fluid and a feed pump (Figure 1). Air cooling is usually preferred as water supplies may not be readily available and air cooling avoids problems with freezing. The cycle is sealed and closed, requiring no makeup fluid. Proper working fluid shall be selected through a parametric analysis in order to reach the highest thermodynamic performance and minimum environment impact for the cycle (Zeki Yilmazoglu, et al., 2014).
An exhaust-heat recovery system can be built up from standard and readily available components. Turbo expanders, for example, are widely used in the gas industry and are available in a range of sizes and operating characteristics. Similarly, the heat exchangers are readily available with several specialist organizations capable of designing and supplying complete organic packages to suit various turbine sizes.
Figure 1: Exhaust-heat recovery system.
The power of the single-stage Rankine cycle may be enhanced by using a cascaded system with an additional stage of expansion in series with the primary expander.
The discharge from the primary expander is put through a regenerator where the residual heat is exchanged with a secondary flow of fluid, which, picking up heat from the regenerator, flows through the second expander before returning to the condenser. This cascaded system can add to the energy recovered from the single-stage expansion process.
The cascaded system described above uses the same fluid for both stages of expansion, but another option is to have two different fluid circuits. The first takes the heat from the exhaust stack and discharges through the regenerator before entering the condenser, and a second separate circuit, using a lower boiling point fluid, takes heat from the regenerator and expands through the second turboexpander. In both cases, the power from the expanders is used in tandem to drive a single electric generator.
Today’s generation of large gas turbines, 25-35 megawatt (MW), being used to drive the gas compressors on pipelines now have levels of efficiency approaching or exceeding 40%. Adding a bottoming cycle can add up to 10% or more efficiency without increasing fuel consumption.
This represents a substantial increase in overall system efficiency. Moreover, the electric power produced can displace a substantial quantity of fossil-fueled power generation. This additional power has the further advantage of being load following, in other words, the power output is in step with demand for flow in the gas pipeline and is available to meet peak demands for electricity, unlike other intermittent sources such as wind or solar.
The exploitation of this technique has taken some time to become practical and widely used. The principal reasons for this are economic, not technical. In considering the viability of each project it is essential that the following requirements are borne in mind:
- The additional capital costs of the plant per kW generated; this will determine payback time.
- Access to the grid for the power produced, distance required to make the connection, and the capacity of the line to match the power.
- Cooperation with the utility which will purchase the power and firm contract agreements for the price to be paid for the power.
Canada Chronology
One of the first examples was completed about 30 years ago at a compressor station in the province of Alberta. The gas turbine was a GE LM 1500, the working fluid was ammonia and the Rankine cycle included a multi-stage axial flow expander in a single circuit.
This unit demonstrated the principle, but performance did not meet expectations. One problem was the lack of uniformity of flow distribution across the exhaust duct. Typically, the exhaust from the power turbine is turned at 90 degrees and discharged into a radial volute before exiting vertically into the stack. This can result in high velocity on one side of the duct and low velocity on the other; the distortion of the flow field in this case did not help the heat-exchanger performance.
This uneven flow distribution is very common and can also result in high levels of noise in the exhaust system. Another difficulty was that the station in question operated with a highly variable load profile; this did allow the exhaust-recovery system to reach optimum conditions for extended periods. The performance shortfall and the complications of servicing a highly toxic ammonia system did not encourage further work.
Contemporary with this work, the Mackenzie Valley pipeline project, proposed in 1977, provided an opportunity for consideration of the organic Rankine cycle for providing power directly to a cooling system. As over half the length of the proposed pipeline traversed the regions north of the 60th Parallel, the gas had to be cooled so as not to melt the permafrost.
Refrigeration would be required at each station in the permafrost region before the hot compressed gas could be returned to the ground. One proposal suggested using a Rankine cycle with propane as the working fluid, heated by the exhaust from the gas turbines to drive the refrigeration compressors.
Design studies showed the feasibility of this concept and pointed out that the recovered energy from the gas turbine unit exhausts would provide sufficient power for this effort. However, the Mackenzie Pipeline project was canceled in 1978 and this proposal was stillborn.
While the concept was never wholly forgotten, there were no practical opportunities to exploit it for several years, until gas prices began rising and concerns were expressed about emissions from power stations. It was not until 1999 that the concept took center stage again when two compressor stations in Alberta, one with TransCanada Pipelines at Gold Creek and the other with the Alliance Pipeline system, were enhanced by waste heat-recovery plants.
Both of the heat-recovery systems were based on a propane cycle and were designed and built by Ormat. Ormat has specialized experience in the design and construction of waste heat and low-temperature heat-recovery systems and was the preferred supplier. The turbine units involved were a Rolls-Royce RB211 at Gold Creek and a GE LM 2500 on the Alliance system. These units are in a similar power band, 25-30 MW, and the single-stage Ormat systems gave about 6 MW power output in each case.
These initiatives were not immediately followed up; time was needed to assess the operational reliability. Following positive results there has been a revival of interest in energy recovery and enhancing efficiency in fuel usage, as the pressure continues to reduce carbon footprint.
In recent years, waste heat-recovery units, again using the Ormat equipment, have been installed on the Northern Borders pipeline system in Idaho, Montana, North Dakota (Figure 2). Additional units have been built in Saskatchewan and Alberta on connecting pipelines. The capability of this technique seems to be assured and the question of future expansion depends upon economics. The environmental benefits of this technology are obvious and significant.
The production of electricity without further expenditure of fossil fuel, no carbon emissions or displacement of power produced by carbon-based generation must be included in the overall positive assessment.
Figure 2: ORC heat recovery unit installed at Northern Border pipeline’s St. Anthony compressor station in North Dakota (Hedman, 2008).
Recovery and Efficiency
The power-recovery potential depends upon the exhaust gas temperature and the mass flow of the turbine. In this respect, some of the older and smaller units, having lower cycle efficiency, show larger proportional benefit than the newer, larger, high-efficiency turbines, such as the RB211.
The Avon, for example, will offer a greater proportionate power recovery than its larger brother. Taking some examples of the results calculated for a number of turbines, using a cascaded heat-recovery system, the possibilities of energy recovery are demonstrated in Table 1. However, it is important to evaluate each case with the proposed units, taking into account the expected loading and use.
Table 1: Exhaust heat-recovery yield for typical gas turbines.
Determining Factors
Before embarking on a waste heat-recovery project the economics must be evaluated. The preliminary study must consider all the relevant economic and operational factors. There are three conditions which must be satisfied at the proposed site:
- • Reasonable access to a robust grid connection.
- • A long-term contract for the sale of the power produced.
- • High load factor on the compressor station.
The first point is essential if excessive connection costs are to be avoided. The second should work to the advantage of the pipeline company and the utility because power will be available all the time the station is running. Point 3 is important to both parties in providing maximum access to green power for the utility and maximizing the cost benefits to the gas company.
A firm, long-term contract for the sale of power generated is essential to ensure payback of the capital and to provide an economic return on investment. A high load factor is important because the income depends on operating hours and the compressor station power output.
The first step in evaluation should be to undertake a survey of all sites to determine the accessibility to the electric grid and, where suitable sites are identified, analyze the current and projected load factors and the potential for heat-recovery output. Given this data, discussions with the utility may lead to establishing the economics of the site and the prospect for mutual interest in proceeding.
In some recent developments, the supplier of the heat-recovery system has undertaken its engineering, installation, maintenance and operation as well as the collection of the revenue for the power produced, and royalty payment to the pipeline company for use of the exhaust heat. This simplifies the risk assessment for the pipeline company and the utility because the supplier of the heat-recovery system assumes much of the risk.
Wider View
This article has concentrated on the recovery of energy and increasing the efficiency of pipeline compressor stations, but we should note the use of ORC energy recovery has wider applications with corresponding benefits.
Distributed generation, with gas turbines close to the power users, requires units of lower power that benefit from ORC heat-recovery application, a subject which is discussed by Welch (2016)1. He demonstrates the efficiency gains and the flexibility of using several small units, both in terms of part-load efficiency and speed of response, an essential feature when backing up the uncertainties of wind and solar power.
Conclusions
The capturing of some of the energy in the exhaust stream from the gas turbine compressor units on a pipeline system and generating electricity is of direct benefit to the efficiency of the electrical grid and does not inflict any penalty on the transmission system.
The extra power provided to the grid is carbon free and “green.” The cost can be competitive with other input sources of environmentally friendly power, such as wind and solar, and has a high availability. The additional pressure loss in the exhaust system of the turbine is small and the loss of power negligible.
While not all compressor stations are candidates, it is estimated there is a significant number on major transmission systems that would qualify and make a significant contribution to sustainable energy. The economics, however, are generally the determining factor.
The key economic parameters to be evaluated in each case include access to the grid and the price to be paid for the power produced. Collaboration between the pipeline company, the utility and the supplier of the heat-recovery equipment is an essential part of a successful project.
In summary, the waste heat-recovery systems can provide electric power equal to or greater than 20% of the power of the base unit without incurring any increase in carbon emissions.
The power produced has high availability in comparison with other renewable sources, is ecologically and environmentally benign, and is load following, that is it will generally track the demand on the electricity system.
References
Hedman, B.A., “Waste Energy Recovery Opportunities for Interstate Natural Gas Pipelines”, Report prepared for Interstate Natural Gas Association of America (INGAA), Washington, DC, USA (Feb. 2008).
1Welch, M., “Flexible Distributed Power Generation Using the Industrial Trent Gas Turbine”, paper presented at the POWER-GEN Europe 2016 Exhibition and Conference, Milan, Italy (June 21- 23, 2016).
Zeki Yilmazoglu, M., Amirabedin, E., and Shotorban, B., “Waste Heat Utilization in Natural Gas Pipeline Compression Stations by An Organic Rankine Cycle”, Energy Exploration & Exploitation, 32, 2, 317–328 (2014).
Authors: Tony Cleveland is president of Cleveland Engineering Services Ltd in Canada, involving in a number of energy-related projects including improvements in energy use and efficiency. His early industry experience includes Bristol aero engines (later Rolls-Royce), and Solar in the U.S. He then joined British Gas (BG) as manager of Machinery Department and was involved in the planning, design and selection of equipment for the BG natural gas transmission system. In 1975, he moved to Canada and worked on northern pipeline development.
Saeid Mokhatab is a gas-engineering consultant who has been involved in several large-scale gas field development projects, concentrating on design, pre-commissioning and startup of processing plants and downstream transmission pipelines. He is a frequent contributing editor to Pipeline & Gas Journal.
Related News
Related News
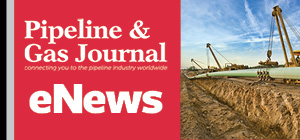
- Trump Aims to Revive 1,200-Mile Keystone XL Pipeline Despite Major Challenges
- ONEOK Agrees to Sell Interstate Gas Pipelines to DT Midstream for $1.2 Billion
- Energy Transfer Reaches FID on $2.7 Billion, 2.2 Bcf/d Permian Pipeline
- Boardwalk Approves 110-Mile, 1.16 Bcf/d Mississippi Kosci Junction Pipeline Project
- Energy Transfer Receives 16 Bcf/d Natural Gas Requests Amid Growing Data Center, Power Needs
- Tullow Oil on Track to Deliver $600 Million Free Cash Flow Over Next 2 Years
- GOP Lawmakers Slam New York for Blocking $500 Million Pipeline Project
- Energy Transfer Reaches FID on $2.7 Billion, 2.2 Bcf/d Permian Pipeline
- Polish Pipeline Operator Offers Firm Capacity to Transport Gas to Ukraine in 2025
- Macquarie, Dow Launch $2.4 Billion Gulf Coast Pipeline Infrastructure Partnership
Comments