October 2017, Vol. 244, No. 10
Features
Does Residual Stress Really Matter in Pipelines?
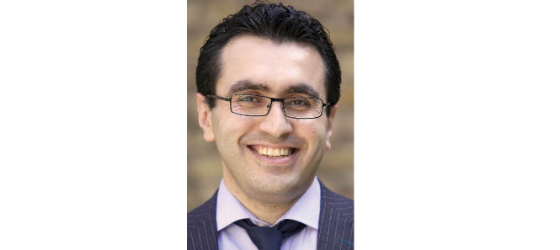
The presence of residual stress in components should not be overlooked, even though it may not be as apparent and quantifiable as primary loads. While residual stress is often responsible for over-conservatism of a design, it can also be considered a major “unknown” contributing factor during a failure investigation.
The origin of residual stress in engineering components is complex and results from manufacturing, processing, fabrication or assembly. Residual stresses exist within a structure and can be either tensile or compressive. Classification is often made based on origin, type or scale, while the fields can be one-, two- or three-dimensional.
Despite the differences in origin, residual stress is always the result of some form of mismatch between different areas within the same part, or even varying phases within the same microstructure. Stresses can be classified as “macro” – residual stresses develop in a component on a scale larger than the grain size of the material – or “micro” in which residual stresses operate over an atomic scale of the material.
Macroscopic residual stresses are often generated in heat treatment, machining, secondary processing and assembly processes, while micro-residual stresses are generated due to a mismatch between phases and constituents, or phase transformations. It is important to note that all types of residual stresses can co-exist in the same component.
Though the presence of residual stress is not as apparent and quantifiable as external loads, it can be determined analytically, numerically through finite element analysis (FEA) or experimentally, using a variety of measurement methods. The choice of one technique over another is based on the sampling volume characteristic of the technique, the types (macro or micro) of residual stresses, the component geometry, materials properties and cost.
Expanding Understanding
The results of a DNV GL UK-led joint industry project (JIP) on the treatment of residual stress in the engineering critical assessment (ECA) of pipeline girth welds under high plastic deformation is now available.
The three-year project, begun in 2009, originated from the requirement to understand the influence of welding residual stress on girth weld integrity of subsea pipelines when undergoing high plastic deformation, such as the reeling process during installation of pipelines. Sponsors included JFE, Nippon Steel & Sumitomo Metal Corp., BP, Petrobras and Subsea 7.
A comprehensive literature review of existing available data was initially performed to understand the redistribution of residual stress under high plastic deformation. This included the following findings:
Manufacturing-induced residual stress – Line pipes of medium to large diameter are often manufactured using the UOE process. Cold forming adopted throughout the UOE process can introduce plastic deformation and significant residual stresses into the finished line pipe material.
Open literature shows that simulation of the UOE manufacturing process by means of FEA is possible and the distribution of residual hoop stress after the UOE manufacturing process suggests that it has no clear trend in its variation around the pipe’s circumference as it is scattered almost randomly.
Published literature also suggests that for smaller hardening moduli, there are important strain/stress localizations during the forming process. As it stands, methods to quantitatively predict manufacturing-induced residual stress magnitude in the hoop and other directions are not detailed in current literature.
Welding-induced residual stress – Welded components are subject to complex transient temperature fields and associated transient thermal stresses, often high in magnitude, around the deposited weld metal and across the structure due to differential thermal expansion/contraction.
These thermal stresses are often high enough to cause yield, introducing misfits into the welded structure, which in turn, generates residual stress when the structure has cooled to ambient temperature.
Materials at the heat-affected zone (HAZ) close to the weld are often subject to cyclic yielding, and the cooling rates associated with welding cycles affect the grain shapes and sizes, including microstructure of the HAZ. Residual stress also exists in welded pipes such as girth welds or seam welds.
Plasticity-induced residual stress – In the offshore industry, pipelines can experience displacement-controlled boundary conditions during installation and operation, such as during pipe reeling, snaking, earthquake or ice impact, giving rise to different levels of primary loads that may induce plasticity and associated residual-stress in the pipe steel structure.
A particularly noteworthy source of plasticity-induced residual stress is from the reeling installation method. The plastic strains experienced by the pipe due to the bending cycles in the reeling process can cause ovality in the pipeline which must be limited, as ovality adversely affects pipeline integrity.
Manufacturing and welding-induced residual stresses can also be redistributed during the reeling process. Hence, when predicting the allowable crack growth during each reeling cycle, one must consider the residual stress redistribution.
Residual stress effects on collapse of pipes – Manufacturing-induced residual stress does not explicitly come into design equations in the current pipeline design codes. The collapse capacity of a pipeline can alter, due to material (Bauschinger effect) and residual stress generated in the manufacturing methods, for example, through the UOE and fabrication process, such as welding-induced residual stress.
The DNV-OS-F101 guideline differentiates the combined loading criteria into the load-controlled condition (LCC) and displacement-controlled condition (DCC), the applicability of the former being more restricted than the latter.
The DCC considers effect of girth weld on the local buckling capacity of the pipeline through the girth weld factor. In comparison, the design equation addressing ovalization buckling due to combined bending and external pressure in the API standard, does not explicitly discuss the influence of welding-induced residual stress or the influence of pipe girth welds in general.
Global buckling may lead to bursting, fatigue, fracture and local buckling. Past research on the effects of residual stress on global behavior of pipelines, for example global buckling, is virtually non-existent. It may be argued that localized residual stress effects, such as girth weld residual stresses, could not affect the global behavior of pipelines. However, residual stress may have an influence on the subsequent limit state, depending on local characteristics.
Residual stress, fatigue and fracture of pipes – Residual stress can significantly affect fracture and fatigue behavior of a structure. One reason for the failure in engineering components in the early days was lack of adequate material fracture toughness. Pipes demonstrate good toughness, hence, ductile fracture is more likely than brittle fracture. However, the environment, such as sour service, can also change the transition region of steel from brittle to ductile failure.
Fatigue and fracture assessment of pipes are usually referred to as ECA. Inclusion of welding-induced residual stress forms a crucial input parameter in ECA. Residual stress is a tensor quantity and varies spatially throughout a weld. However, it is common practice to use simplified residual stress profiles in either the weld longitudinal or transverse directions in ECA procedures.
The R6, BS 7910, API 579-1/ASME FFS-1, FITNET and SINTAP guidelines provide various upper-bound residual stress profiles that can be considered in pipe girth weld ECA. The residual stress profile recommended by BS 7910 is one of the simplest and often leads to the most conservative results.
The DNV-OS-F101 recommendation on ECA assessment is mainly based on BS7910. Figure 1 show various code recommendations for a pipe with a fixed OD and fixed welding heat input with through thickness welding transverse residual stress. It highlights major differences in recommended upper-bound distributions based on various codes and open literature. (Fig. 1)
Figure 1: Through thickness as-welded transverse residual stress for a girth weld per various codes at a specific heat input.
To produce more realistic acceptable defect sizes in fracture assessment of pipelines, both strength mismatch condition and welding residual stress effects should be addressed thoroughly as they are inherent properties of a girth weld.
It is common knowledge that residual stress can affect the fatigue behavior of a structure as outlined in DNV-RP-C203. There are some processes (thermal or mechanical) for producing beneficial compressive surface residual stresses for enhancing fatigue life of a structure.
Influence of residual stress on fatigue is addressed quantitatively by superposition of applied stress intensity factors with secondary stress intensity factors. To allow for the potential presence of highly tensile welding residual stresses, the fatigue crack growth curves for fatigue stress ratio more than 0.5 are recommended in DNV-OS-F101 and DNV-RP-C203. However, there is no detailed guideline to estimate residual stress re-distribution due to fatigue crack growth.
Methodology
Following comprehensive review, various FEA models were developed to predict the welding residual stress redistribution in a girth welded pipe in which the outer diameter of the pipe and the wall thickness matched the measured pipe geometry from the requirements of the JIP. Several detailed measurements of residual stress and FEA studies were done in this JIP:
Preparing and welding typical offshore seamless pipes, in a similar practice done in the offshore industry.
Full-scale reeling tests at DNV GL’s laboratory facilities at Hovik, Norway.
Surface and through-thickness measurements in as-welded condition and after full-scale reeling tests.
FEA of full-scale reeling simulation.
Comparisons between FEA and measurements to understand re-distribution of residual stresses at different circumferential clock positions of a girth weld. (Fig. 2 & Fig. 3)
Figure 2: The full-scale testing set up to simulate reeling process at DNV GL’s laboratory.
Figure 3. Comparison of the predicted through thickness axial residual stress from the FEA against the measurement after pipe reeling.
Main Findings
There is no evidence that the current DNV-OS-F101 guidelines are overly conservative or non-conservative for treatment of residual stress in pipeline design.
Both the numerical and the experimental test results in the DNV GL-led JIP have confirmed that reeling substantially reduced the welding residual stresses at locations at a distance from the neutral axis. However, welding residual stresses close to the neutral axis were not relaxed by reeling, which may affect the integrity of the pipeline when it is subjected to lateral loading during operation, for example, through lateral buckling. The level of plasticity-induced residual stress close to the neutral axis was also found to be significant.
There are uncertainties associated with the residual stress in an ECA, which seems due to an assumption of upper-bound residual stress and strength mismatch between welds and parent metals. Residual stress redistribution owing to mechanical overload is likely to be dependent on the nature of the stress tensor and how it is distributed.
Although peak value of through-thickness residual stress in pipes is reduced following high-plastic deformation, any further reduction compared to those allowed by ECA codes should be done cautiously as the over-conservatism of residual stress recommendations in the ECA codes compensate others under conservative parameters.
Material properties and welding strength mismatch level can affect the initial welding residual stress and redistribution of their profiles under primary loading. Redistribution of mismatched weld residual stress profile is generally determined by the mismatch condition, whereas tensile hardening will determine their magnitude.
Conclusion
The influence of residual stress on pipeline integrity depends not only on the length scale, magnitude, direction and type of the residual stress, but also the “limit state” in pipeline-design consideration. The extent of such influence is also affected by the material property of the pipeline, the manufacturing method and thermal history. Figure 4 summarizes the different factors that should be considered when answering the question, “Does residual stress matter in pipelines?” (Fig. 4)
Figure 4. Factors to consider when assessing influence of residual stress on pipeline integrity.
Large variations in welding residual stress profiles are known to exist. However, available data on welding residual stress related to girth welds of offshore pipelines is limited and the need is significant, requiring further study to raise the confidence level on this challenge in the pipeline industry.
There is also limited measured residual stress data for carbon-manganese steel pipes, even fewer for clad, lined and super-duplex pipes. Very few studies have been reported on the influence of residual stress on pipeline sour service.
That said, the presence of residual stress in components should not be overlooked, even though it may not be as apparent and quantifiable as primary loads. Though techniques for measuring residual stresses have become more practical in recent years, the application of such is still limited, prompting the need for further investigation.
Comments