March 2013, Vol. 240, No. 3
Features
Battelle Acts On Corrosion Detection And Prevention
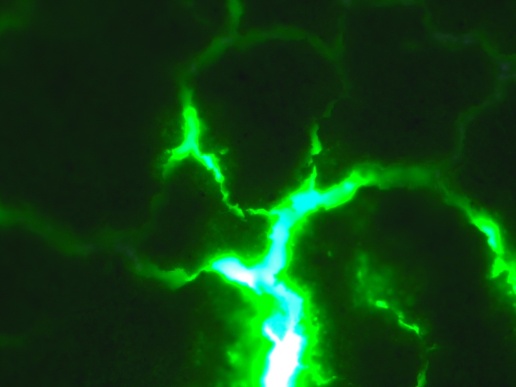
Corrosion is a widespread problem across many industries. Corrosion-related costs to the pipeline industry are estimated anywhere from $5.4 billion to $8.6 billion annually in a report by NACE and CC Technologies. These costs include failures, capital, and operations and maintenance.
Improved methods of detection, as well as improved corrosion prevention coatings, can help reduce these costs. New technologies such as self-repairing coatings reduce the need for field repairs or extend the intervals between repairs.
Coatings are widely used for corrosion prevention on oil and gas pipelines. Battelle has a number of new developments in the detection, evaluation, and prevention of corrosion. Battelle continually builds on experience across multiple market sectors to develop new technologies for corrosion control. Some of the examples presented here were originally developed for bridges, aircraft, or marine use but the technology can be transferred to pipeline use.
Corrosion Modeling
Battelle has two modeling efforts related to corrosion. The first is the Environmental Severity Index (ESI). This model uses data on temperature, humidity, chlorides concentration, and UV radiation intensity to predict corrosion rates for both ferrous and non-ferrous alloys. Battelle has collected sensor data from more than 2,000 sites worldwide. Predicted corrosion rates are shown in Figure 1.
Rates for a number of locations have been verified experimentally. Battelle’s facility in Daytona Beach, FL, shown at the top of Figure 1, is one of the most corrosive marine environments available. Sensors deployed in various locations also allow real time assessment of conditions for corrosion prevention or monitoring. These sensors do not directly measure corrosion of a structure, but they do provide information on the corrosive severity of the local environment, which can serve as a leading indicator for corrosion.
The second type of modeling incorporates corrosion damage into mechanical models. Once the rate of corrosion and type of corrosion occurring are known, this information can be included in mechanical models. Damage tolerant corrosion modeling applies fracture mechanics to corrosion.
Custom algorithms developed by Battelle include the effect of corrosion in Finite Element Analysis (FEA) by treating corrosion pits as cracks. The custom algorithm predicts how the cracks will grow, permitting predictions of service life based on structures and loads and environmental conditions. Testing shows that corroded structures have shorter lives and lower failure stresses. The new models incorporate this effect and accurately predict real-life behavior.
Figure 1. Predicted corrosion rates for many locations, sorted by severity.
Non-Destructive Imaging For Detection
Battelle is working with a range of technologies for non-destructive evaluation of coatings and corrosion in pipelines and other applications. Corrosion underneath a coating often cannot be detected by visual means until the coating fails. Early detection allows for timely mitigation or repair of corrosion damage.
Ultrasonic inspection techniques have been in use for several years. Ultrasonic inspection will detect cracks and significant changes in wall thickness due to metal loss. However, depending on the resolution of the system, ultrasonics may miss early surface corrosion. Battelle has been working on an alternative non-destructive evaluation approach using Terahertz (THz) imaging.
THz imaging has been found to be effective at imaging corrosion through intact coatings on metal surfaces. This method uses THz electromagnetic waves, which fall between infrared and microwave radiation on the electromagnetic spectrum. Many organic coatings are transparent to THz radiation while metal substrates will reflect an incoming signal. The technique has been demonstrated for early detection of corrosion on aircraft. High-resolution laser scanning also has been demonstrated for corrosion detection. This technique does not penetrate the coating, but picks up subtle defects caused by the underlying corrosion.
Corrosion Prevention Coatings: Primers And Top Coats
Many traditional corrosion preventive compounds used in coatings are based on hexavalent chromium and other toxic metals and compounds. However, the new restrictions of hazardous substances (ROHS) requirements implemented in 2006 have limited the use of hexavalent chromium. Battelle evaluated a variety of non-chromium surface pre-treatments for metals. The Prekote™ surface pretreatment is a silane based treatment while AC-131™ is a sol-gel treatment. Both of these are used in place of chromium, cadmium and other toxic pretreatments used on metal surfaces before painting.
Advanced primers also are replacing hexavalent chromium. Battelle developed a series of ferrate compounds for corrosion prevention and incorporated them into existing MIL-spec primers without corrosion prevention compounds. Samples with these ferrate compounds are undergoing exposure at Battelle’s Florida Marine Research Facility (FMRF). After three years of continuous exposure, the ferrate compounds are performing as well as hexavalent chromium compounds, and far better than other hexavalent chromium replacements.
Battelle also has developed an Extended Life Topcoat (ELT) or Advanced Performance Coating (APC) based on polyurethane and fluoropolymers that is used by the US Air Force to prevent corrosion of aircraft. The topcoat is resistant to degradation from aging and exposure to the elements and will last longer than traditional topcoats. The topcoat has been qualified to MIL specs and is produced commercially by an existing coatings supplier. This has the advantage of extending the maintenance intervals, reducing the frequency for repair or replacement of the coating.
Self-Healing Smart Coatings
Corrosion-prevention coatings inevitably will suffer damage over their lifetime. When damage is detected, the coating can be repaired or replaced. The traditional approach has been to wait until visible damage has occurred, such as visible cracks, blisters, or peeling of the coating, or a raised surface caused by corrosion of the substrate. However, a significant amount of damage to the substrate can occur before this visible evidence appears. One approach is to use the non-destructive imaging capabilities discussed above. As an alternative, Battelle has developed a novel type of smart coating that incorporates both self-healing and damage indicator functionalities.
Numerous reports of self-healing coatings have appeared in the literature in recent years. A common technique is to use micro-encapsulation techniques to encapsulate a thermosetting resin inside a thin shell. These microcapsules are dispersed in the coating along with a catalyst for the thermosetting resin inside the microcapsules. When the coating is damaged, capsules rupture at the damage site, and the catalyst initiates curing of the resin as it flows into the damage zone.
There are a few challenges with this approach. The catalyst must not react with the original coating resin. It must also be stable over many years so that it can still react with the healing resin inside the microcapsules when damage occurs. Enough catalyst must be present to ensure a good cure of the healing resin because the resin won’t start curing until it encounters the catalyst.
Battelle’s self-healing smart coating uses a novel variation on micro-encapsulation. Rather than using a thermosetting resin, a thermoplastic resin is encapsulated. When damage occurs, the capsules break and the resin flows out and into the crack. Unlike a thermosetting resin, the thermoplastic resin does not need to encounter catalyst to heal the coating. Catalyst stability and compatibility with the host coating is no longer an issue.
Battelle has tested both acrylic oligomers and polymers as the thermoplastic healing resin. The oligomers had lower viscosity and filled the cracks better as a result. Improved healing was demonstrated by reduced corrosion in test panels exposed to a standard corrosive environment. Control panels had the most corrosion at induced flaws, panels with polymer healing agents were in the middle for performance, and panels with oligomer healing agents had the least corrosion.
In addition to improved healing characteristics, Battelle’s smart coating incorporates a damage indicator compound. Molecules of 8-hydroxyquinoline (8-HQ) are tethered to the outside of the capsules during the micro-encapsulation process, as shown in Figure 2. When corrosion of aluminum or steel occurs, the metal ions form a complex with the 8-HQ. This complex fluoresces under ultraviolet light, making corrosion visible by inspection with a handheld UV lamp, as shown in Figure 3. The complex also has high contrast to THz imaging, improving corrosion detection with THz imaging methods discussed earlier.
Figure 2. Battelle’s approach to a smart coating compared to a traditional smart coating.
Figure 3. The smart coating fluoresces in cracks under UV light.
Chrome Reduction In Coating Removal And Maintenance
As noted earlier, new primers and coatings have replaced toxic hexavalent chromium with alternative corrosion prevention compounds. However, older structures often have primers with hexavalent chrome. Performing maintenance on these structures, including stripping and repainting, can expose workers to toxic dust or release hexavalent chromium into the environment. Battelle has developed a process for converting hexavalent chrome into less toxic trivalent chrome. The reducing material can be mixed with liquids, incorporated into wet sanding, or wiped onto the coated surface. A color change indicates that the process is working. Reductions as great as 99.9% have been observed. This improves worker safety and reduces harm to the environment.
Corrosion Testing And Evaluation
A wide range of technologies and methods are used for testing and evaluation of corrosion and corrosion prevention coatings. One popular option is real-time outdoor exposure. As noted earlier, Battelle’s FMRF provides one of the most corrosive natural environments available in the U.S. The environment is classified as “Humid Subtropical” by the National Oceanic and Atmospheric Administration (NOAA), with an average temperature of 22°C (72°F) and an average relative humidity of 78%.
The oceanfront property has a high site surf intensity resulting in levels of atmospheric chlorides averaging 200 mg Cl/m2/day, contributing to the aggressive corrosive environment. Marine exposure for biofouling studies is also available from docks along the Halifax River estuary.
In cases where outdoor exposure is not practical, or accelerated testing is desired, a variety of indoor exposure systems are available. Coated samples can be exposed to salt fog, acidic salt fog, or continuous aqueous immersion, with periodic visual evaluations of corrosion according to ASTM and other standard methods. Prohesion is a modified salt fog test that alternates salt spray with drying cycles. This creates a more aggressive corrosion environment. Samples can be scribed to create controlled flaws in the coating, and the corrosion creep away from the scribe can be measured. Exposure chambers with an ultraviolet light (UV) component, such as QUV cabinets and the Atlas Weatherometer, simulate both daylight and rain cycles in an outdoor environment for realistic accelerated weathering of coatings.
Another common laboratory test for the durability and effectiveness of corrosion prevention coatings is Electrochemical Impedance Spectroscopy (EIS). An RC circuit is created between an electrode in the conductive liquid on top of the coating and the metallic substrate under the coating. Equivalent circuit analysis is used to model the degradation behavior of the coating and allow measurement of instantaneous corrosion rates of the substrate. Tests can be run for short times as a screening tool for coatings. Longer tests, weeks to months in duration, can be used to predict the long-term effectiveness of coatings.
Conclusions
New technologies are reducing the cost and impact of corrosion. Corrosion can be monitored and predicted based on the local environment, allowing for adjustments to maintenance schedules. Improved detection methods find corrosion before it’s visible to the naked eye. New coatings and additives resist corrosion and extend the life of coatings, reducing the need for repainting while being friendlier to the environment. Corrosion cannot be eliminated completely, but it can be managed effectively.
Comments